Home » Research projects
Category Archives: Research projects
Irene at WHOI
Irene’s doctoral thesis investigates the effects of decreasing oxygen conditions on microbial communities through the aerobic respiration kinetics and how these communities adapt at the oxic-anoxic interfaces. As a part of her PhD work, Irene has participated in two oceanographic expeditions to oxygen minimum zones (OMZs) in the North and South Pacific Ocean. During these campaigns aboard scientific research vessels, she performed on-site experiments and collected samples for molecular biology analysis, aiming to understand microbial behaviour at the genetic level.
Currently, Irene is undertaking a predoctoral research stay at the prestigious Woods Hole Oceanographic Institution (Massachusetts, USA) from September 20 to December 20, 2024, under the supervision of Maria G. Pachiadaki, an expert in molecular biology, particularly in metagenomics and metatranscriptomics of marine environments. During this stay, Irene is conducting DNA and RNA extractions to describe microbial communities, as well as identifying key genes and enzymes involved in their adaptation to decreasing oxygen conditions. This research sheds light on how microorganisms adjust to oxygen-depleted oceans, where critical biogeochemical cycles and functioning of the world’s oceans depend heavily on microbial processes.
The effect of an invasive algae on the beach biogeochemistry
We have successfully completed the second year of field samplings of the project “Beach biodiversity and the ecological role of the invasive species Rugulopteryx okamurae in beach ecosystem functioning” Project Excel_01050, lead by Ivan Franco Rodíl.
During the second year, we contributed in measuring the bacterial diversity and leaching of dissolved organic carbon associated with accumulation of the invasive algae on different beaches of the coast of Cádiz.
The samples are currently being analysed but they show already how the organic matter of the beach racks alters the microbial benthic community along the beach transect. Hopefully the results will contribute to the management of this environmental issue that affects the local economy (fisheries, tourism).
Effects of heatwaves on the physiology of benthic microalgae
Andalusia has a high diversity of wetlands. These include intertidal shallow systems, which are important both ecologically and economically, given that the microalgae that grow at the sediment surface are an important source of food for higher-level organisms such as fish, oysters etc.
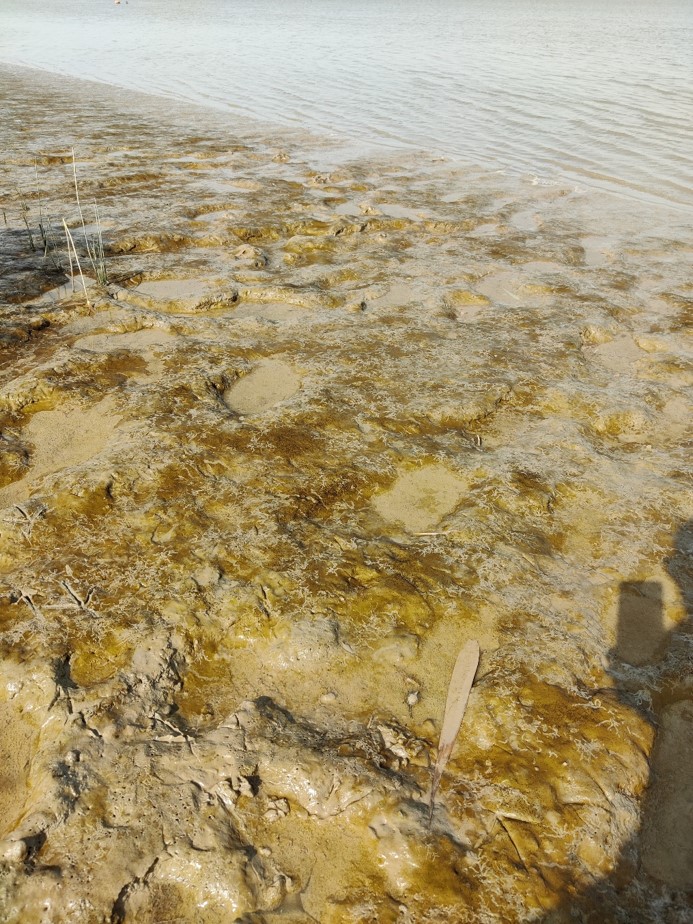
The microphytobenthos (MPB) is the photosynthetic community living on the sediment surface. They play an important role in shallow estuaries as they can be responsible for a large part of the primary production. This community is dominated by diatoms (algae covered in a silicate casing, called the frustule) and can achieve very high densities giving a golden colour at the sediment surface.
In order to deal with the highly fluctuating environments conditions that characterize the intertidal areas, such as light, temperature, tide cycle and seasonal variations, diatoms have developed several strategies. For example, they have the capacity to migrate vertically during the day to adapt to the light conditions and tidal variation.
Marika Mecca, one of our PhD students, is trying to understand the effects of climate change on the physiology and behavior of these benthic microalgae. Under normal conditions, the sediment surface undergoes large changes in temperature between night and day, under water (immersion) or exposed to the air (emersion), and between seasons. Thus, under a climate change scenario where heatwaves are more frequent, the shifts in temperature will be even larger and the effects on the physiology of the microalgae can be detrimental.
To study these effects, Marika uses various instruments and sensors in the lab, such as oxygen microsensors to measure the amount of oxygen produced, temperature sensors to measure the variations vertically, reflectance sensors to detect the migration rhythms etc.
The principal area of study is the Guadalquivir River. Once the cores are collected in the field, back at the laboratory these sediments are subjected to different conditions simulating the changes observed in the field under normal and extreme weather conditions.
The results show an effect of prolonged high temperatures on the migration rhythm of the microalgae (avoidance of the sediment surface), although differences in total net metabolism are less probably due to a stimulation of rates by the higher temperature. These results were presented at the ASLO 2023 conference in Mallorca.
Annual Sampling in the Bay of Cádiz
Within the framework of the EXTREME-FUN project, we are investigating how benthic primary production, the biomass of microphytobenthos and the amount of carbon that is buried or exported from the system varies throughout the year, and at different points in the Bay of Cadiz.
How do we do it?
Using a zodiac, which allows us to access difficult shallow areas, we collect 25 sediment cores distributed throughout the Bay and 15 more distributed in each of two opposite areas with different characteristics (one sandy, one muddy), allowing to obtain a higher spatial resolution.
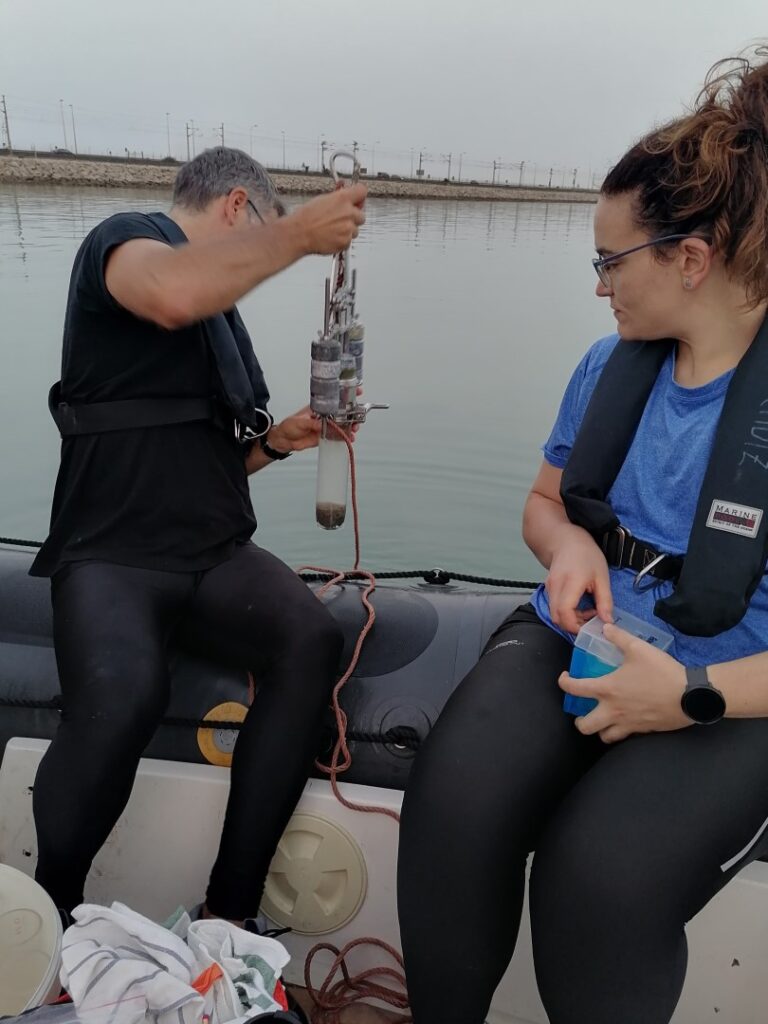
In order to scale up the data obtained to a higher spatial resolution, we fly at the same time drones equipped with multispectral and thermal cameras and we coordinate the sampling with the pass of SENTINEL 2 satellite.
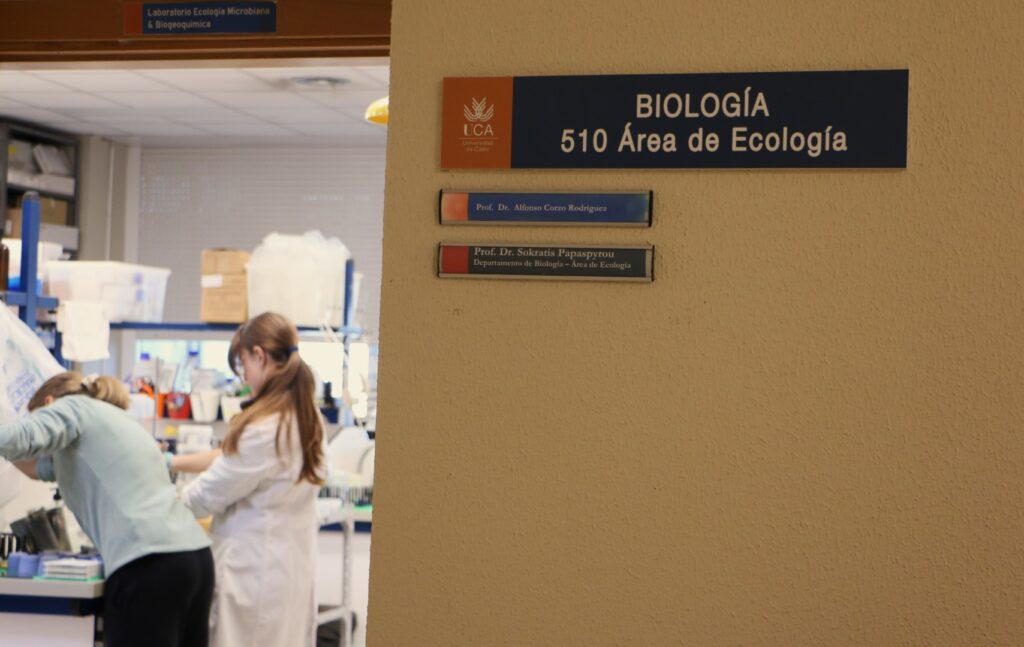
When the sampling is finished, we transport all sediment cores to the laboratory and we measure several biogeochemical variables such as oxygen fluxes, reflectance, chlorophyll, grain size, etc.
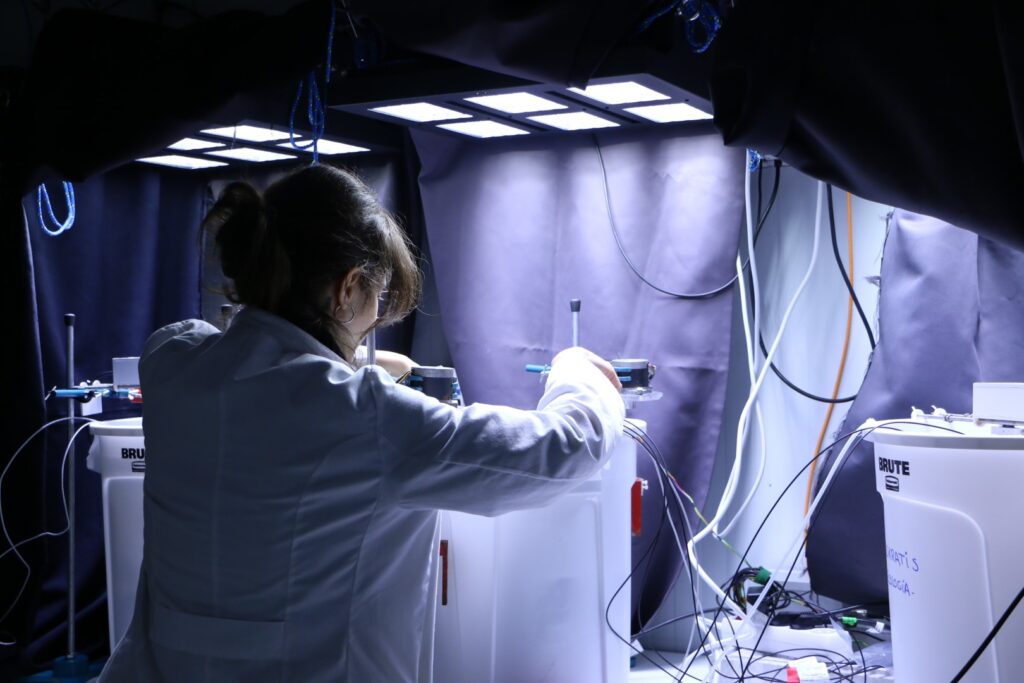
¿Why are we doing that?
In order to estimate the effect of extreme events, such as heatwaves and storms, which are becoming more frequent and intense, on the biogeochemistry of intertidal sediments, it is essential to know the baseline conditions in the Bahia. Therefore, this sampling intends to increase the data available, allowing us to upscale using remote sensing, before studying the extreme events.
By : Sandra Rizzo Calderon
Unveiling microbial strategies in a changing ocean: microorganisms adaptations to decreasing oxygen conditions
A brief introduction…
Oceans deoxygenation is considered one of the most important threats currently occurring in marine ecosystems, as the oceans have lost about 2% of their oxygen inventory over the last 50 years. However, the impact of deoxygenation is not only happening in oceanic zones, hypoxic and anoxic coastal zones are also increasing. To get an idea of the areas of low oxygen content, here is a global map from Breitburg et al. (2018). The red dots refer to hypoxic coastal zones (dissolved O2 content < 60 µmol O2 kg-1), while the shaded blue areas represent oxygen minimum zones (OMZs).
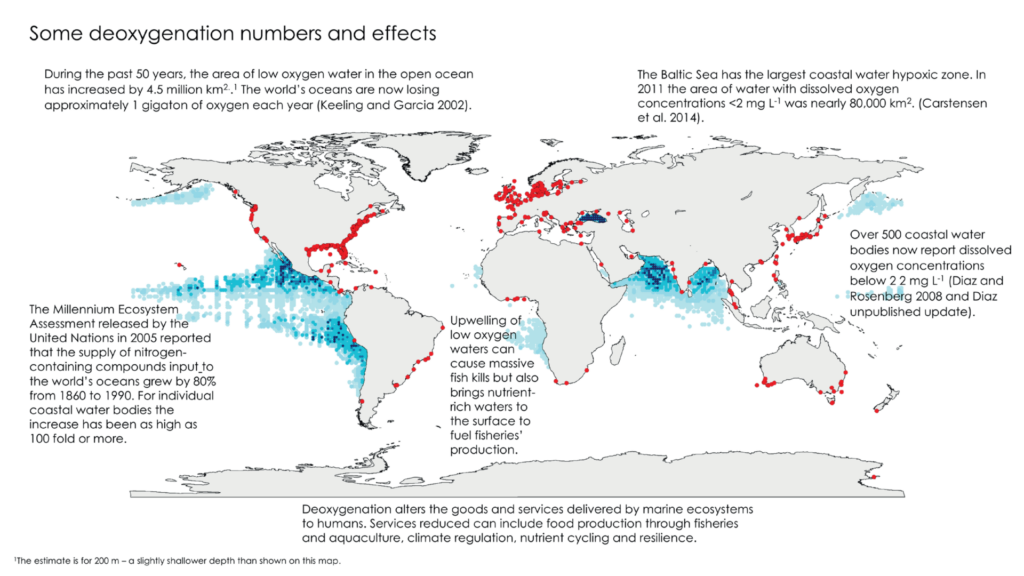
Consequently, microorganisms have to face these decreasing oxygen conditions, but how do they do it?
Aerobic respiration is carried out by terminal oxidases, a group of enzymes that play a key role in the final stage of aerobic respiration. There are two groups of terminal oxidases: low and high affinity terminal oxidases (LATO and HATO), with a half-saturation constant (Km) of 200 nanomol L-1 O2 and 2-8 nanomol L-1 O2, respectively. In contrast to eukaryotes that only possess low-affinity terminal oxidases, prokaryotes have both high- and low-affinity terminal oxidases. Prokaryotes can use both types during aerobic respiration to modulate their affinity for oxygen, and therefore, adapting to decreasing oxygen conditions.
Michaelis-Menten kinetics model (picture below) can be used to study the effect of oxygen concentration on respiration rates and thus, the microbial performance under these decreasing oxygen conditions. The kinetics parameters, maximum respiration rate (Rmax) and half-saturation constant (Km), offer insights into the relative use of different oxidases and overall adaptation strategies employed by microorganisms.
As the extension and distribution of low-oxygen zones are increasing, their impact on global biogeochemical cycles needs a deeper understanding of microbial processes. Therefore, aerobic respiration kinetics in environments with large oxygen gradients will help us better comprehend the distribution of microorganisms and their microbial processes occurring at the boundary of oxic-anoxic conditions.
Oxygen Minimum Zones: an overview
Oxygen minimum zones are systems where sinking of organic matter produced by primary production at the surface coupled with slow water circulation leads to the formation of oxygen-deficient water masses. The strong stratification observed in tropical areas allows these OMZs extend over vast regions, covering over 30 million Km2, which means 8% of the total ocean surface. This highlights the significant role of these zones on shaping the inhabiting marine ecosystems and biogeochemical cycles but, how?
OMZs play an essential role in the global nitrogen cycle, involving several chemical species and different bacteria processes. OMZs are associated to denitrification, a process that only occurs in oxygen-deficient regions. This process convert nitrate (NO3–), one of the main nutrients in the ocean, into gaseous nitrogen (N in the form of molecular nitrogen, N2, or nitrous oxide, N2O), which is lost to the atmosphere and contributes to the oceanic nitrate deficit.
OMZs are not only involved in the nitrogen cycle, but also in another biogeochemical processes like the production of production of sulphide (H2S) and methane (CH4), and limitation of atmospheric CO2 sequestration by the ocean.
However, the impact of OMZs extends to biodiversity, as this oxygen-deficient zone can serve as a refuge from predation for organisms specially adapted to these oxygen levels.
For this reason, OMZs are crucial for studying aerobic respiration kinetics. This natural gradient of oxygen concentrations allows us to investigate and study how different microbial communities adapt to different oxygen levels within the water column. By mimicking these gradients in controlled incubations, we can observe how respiration rates change across different oxygen concentrations, making OMZs a natural laboratory for understanding aerobic respiration kinetics.
Study of aerobic respiration kinetics
In order to measure the respiration kinetics, we perform a series of incubations in modified glass bottles. We monitor and follow the oxygen concentration throughout incubation time with a set of high-resolution oxygen sensors to measure oxygen consumption rates from anoxia to full oxygen saturation. The incubation bottles are kept in dark and placed in a temperature-controlled bath. The set-up of the experiment is shown below to the right.
Using the changes in the oxygen concentration during the incubations, we can calculate the respiration rates from linear regression of O2 concentrations over time in the different bottles with different oxygen concentration levels. We then use all the respiration rates measured at the different oxygen concentrations for fitting the Michaelis-Menten model and characterize the respiration kinetics of the microbial community, as we can see in the following graph.
The points in the graph represent the respiration or oxygen consumption rates across the wide range oxygen concentration used, from very low oxygen values to almost full oxygen saturation. For a better visualization of this wide range we also show the same data but using logarithmic scales on both oxygen concentration and respiration axes, showing the fitting of the data to the Michaelis-Menten model across the wide range of oxygen concentrations used.
The work behind the incubatios
Prior to running the incubation experiments, is essential to understand the water column’s physical and chemical characteristics. For that purpose, we use a conductivity-temperature-depth sensor, more known as CTD. This instrument is widely used in oceanography to characterize the changes of conductivity and the temperature with depth. Modern CTDs are also equipped with additional sensors to measure and collect data from other parameters like oxygen, fluorescence (indicative of phytoplankton activity), turbidity, etc.
The CTDs are attached to a metallic structured called rosette, which holds a bunch of sampling water bottles that are used to collect water from different depths. These bottles can be controlled remotely from a control room, where scientists receive live data from the CTD and can monitor changes in the physical and chemical characteristics of the water mases as the rosette descends. Once the rosette returns to the surface, bottles can be closed remotely at the selected depth. Here is an example: a rosette from the R/V Atlantis (Woods Hole Oceanographic Institutions, Massachusetts, E.E.U.U.). This rosette is composed by 24 sampling water bottles of 12 L each. However, the number and size of bottles can vary depending on the specific rosette design and research requirements. The CTD sensor is placed below the set of bottles. As a safety measure, the rosette is always securely tethered to the floor.
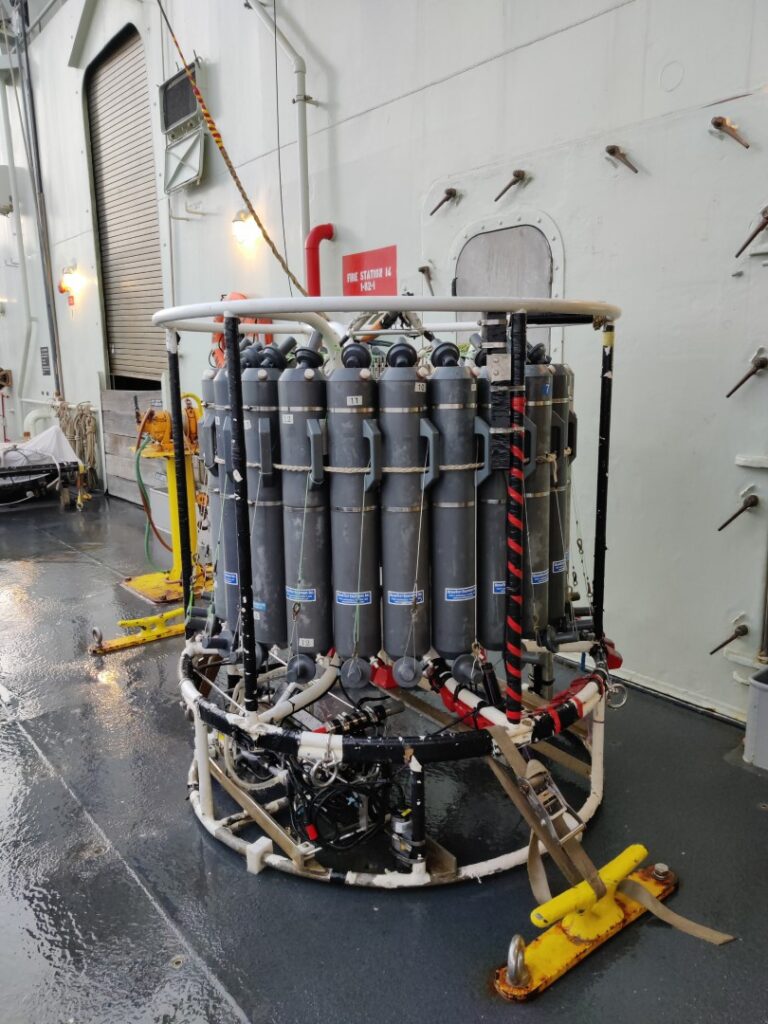
The process of the CTD deployment and recovery can be carried out by the scientific team on board with guidance and indications of the technical staff, or remotely controlled by a technician, as it’s shown in the pictures below. On the other hand, scientist receive the real-time data from the CTD sensors and the other sensors associated, obtaining the water column profile. Based on that, scientist make decisions regarding the following experiments and samples collection. Once the rosette is back to the surface and secured, water is directly collected from the bottles, as they have a spout for easy retrieval by directly connecting a tube. The success of samples collection lies on collaboration between scientist and the technical staff!
Oxygen measurements in the lab
Oxygen measurement
Oxygen is the most advantageous electron acceptor during the process of respiration. That is why all higher organisms use oxygen to breathe. The oxygen is also involved in the re-oxidation of most reduced compounds produced by other types of anaerobic respirations (e.g. H2S, Fe2+, Mn2+). In addition, during daytime, photosynthesis by benthic microalgae produces oxygen Therefore, by quantifying oxygen consumption rates, we can have a net measure of both aerobic and anaerobic respiration processes, and of photosynthesis during light, occurring in the sediment.
How do we measure it?
In our laboratory, we usually use two different type of techniques, one using microsensors to measure vertical profiles of oxygen across the sediment water interface and with depth in the sediment and another by measuring the changes in oxygen concentrations in closed core incubations over time.
These methods provide similar but complementary information.
Microsensors
Profiles made with microsensors provide important information as to the total flux of oxygen across the sediment water interface, but also to the deeper layers, the depth of maximum oxygen production in light corresponding to the layer of microalgae, and the depth of the oxic zone (when oxygen concentration goes to zero). However, given that this method is based on a microsensor, fluxes measured are solely diffusive (movements of oxygen at a microscale) and are very localized to a small area around the tip of the sensor (a few microns).
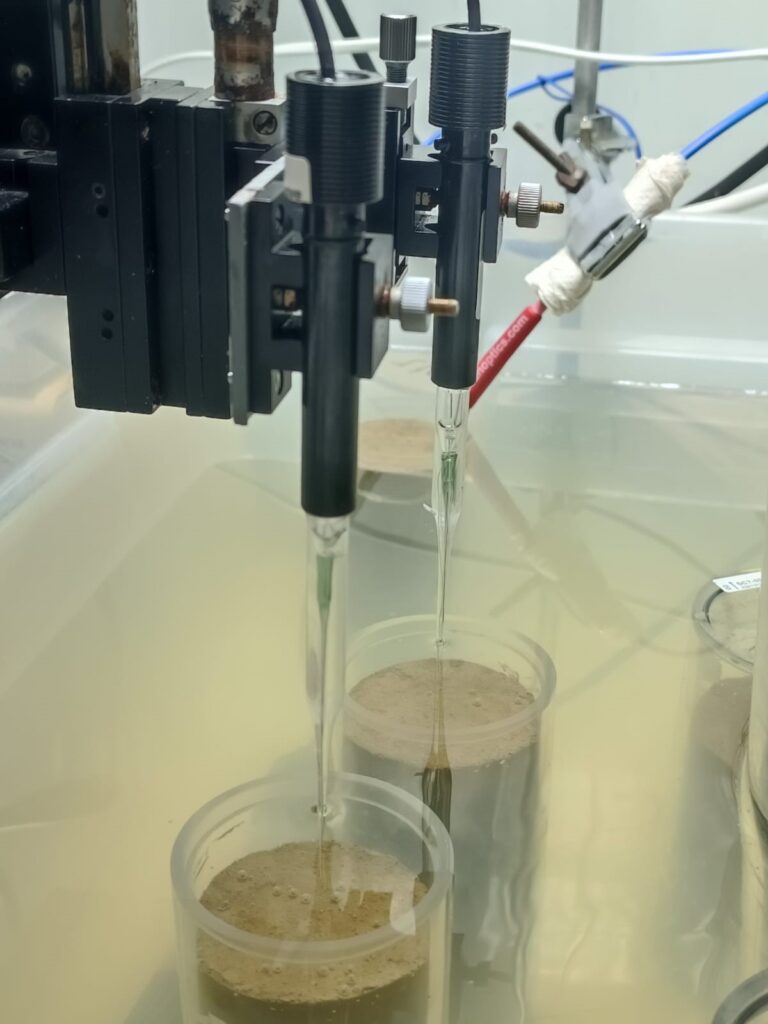
Whole core incubations
Whole core incubations and measurements of the oxygen changes, provides a more integrated estimation of the oxygen flux as it includes a larger area, the consumption due not only of the microorganisms but also of larger macroorganisms (worms, molluscs, crustaceans etc). It also allows to include the effect of another process, that of advection that moves the oxygen molecules at larger spatial scales (for example by waves, the ventilation of burrows etc). This is why, fluxes measured by whole core incubation are called sometimes total fluxes.
To do this, we close the core (cylinder) containing our sediment ecosystem. On the inside of the lid there is a gel that contains a special dye that reacts with oxygen. Using an optical fibre we can measure through the transparent lid the changes in the fluorescence of the compound as the oxygen concentration changes inside the core. Quite cool..
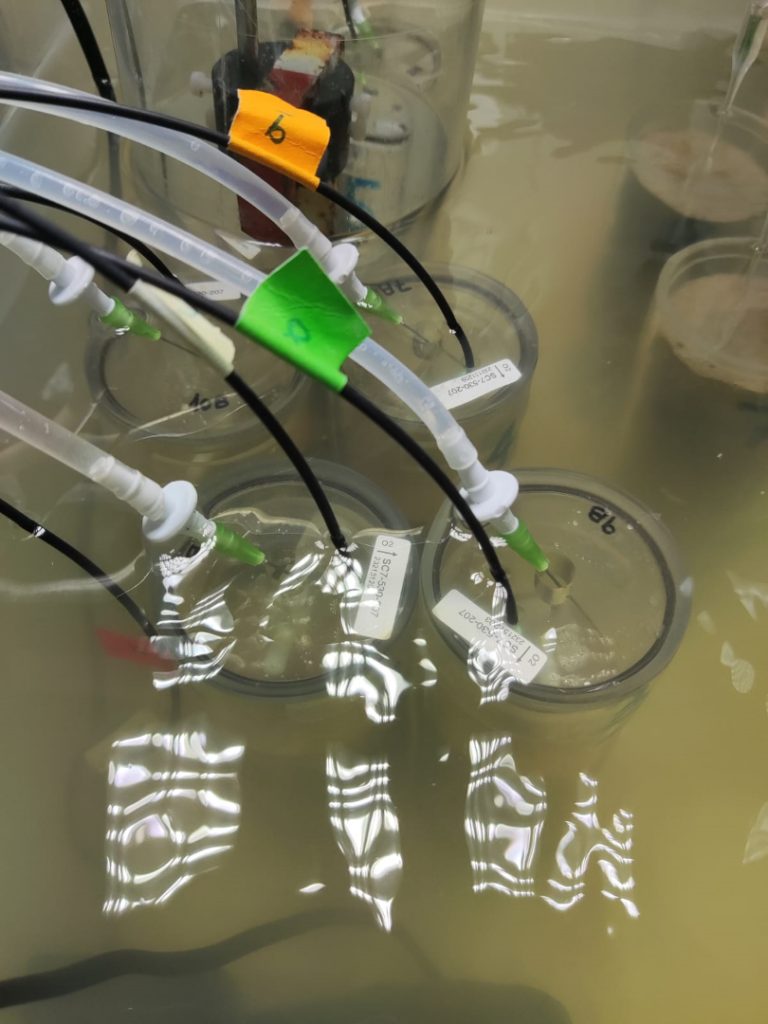
However, both of them allow us to study the sediment metabolism and depending on the specific aims of our experiment, we use one or the other, or both. The more tools, the better!
Biocobre: new project at MEBL
The research group in Microbial Ecology and Biogeochemistry of the UCA (MEB-LAB) initiates the development of a new line of research on copper precipitation in environments contaminated by acid mine drainage in the framework of the project “Bioprecipitation of metallic copper from acid mine drainage in the Iberian Pyritic Belt” BIOCOBRE, funded by the Junta de Andalucía.
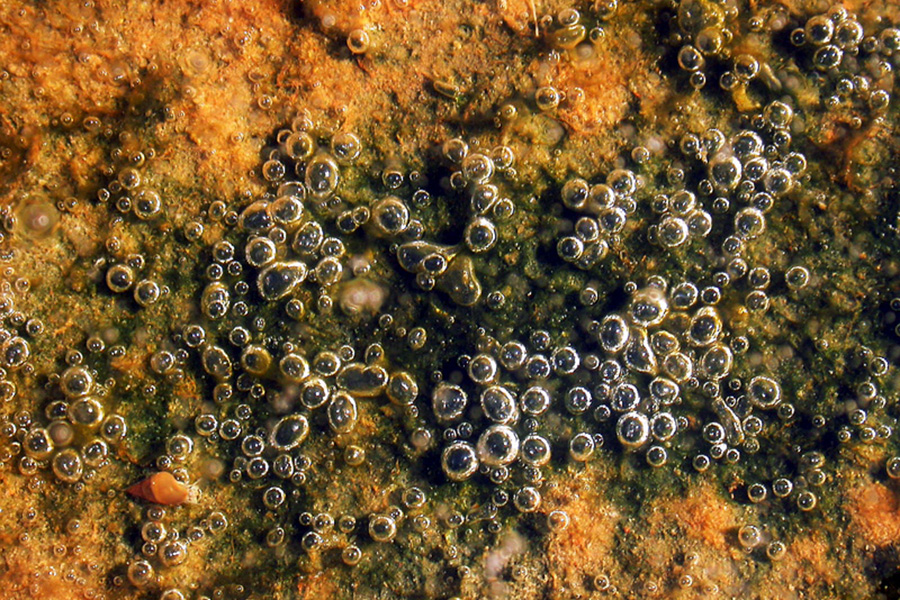
The objective of the project is to study precipitates of copper salts and oxides in environments contaminated by acid mine drainage (AMD). The biogeochemical conditions, the microbial community and the metabolic pathways involved in the precipitation of copper nanoparticles in biofilm growing in acid mine drainage (AMD) areas in the Faja Piritica Iberica (Huelva) will be investigated.
Precipitates of Cu2+ and Cu+ salts and oxides are common in acid mine drainage (AMD) contaminated environments, but Cu0 precipitates are not. BIOCOBRE will investigate the biogeochemical conditions, microbial community and metabolic pathways involved in the precipitation of metallic copper (Cu0) nanoparticles in a biofilm growing in the acid mine drainage (AMD) of the abandoned mine Mina Esperanza (Huelva). To understand the Cu0 bioprecipitation process, we will set the following objectives: 1) define the geochemical environment within the biofilm where Cu0 precipitates and accurately measure Cu0 precipitation rates under different conditions, 2) describe the evolution of the biofilm microbial community from colonization to maturity under different environmental conditions, 3) isolate and culture the biofilm microorganisms (mainly fungi and bacteria) potentially involved in the Cu0 precipitation process. To achieve these objectives, we will use a multidisciplinary approach in which we will combine state-of-the-art techniques from different scientific disciplines: microsensors (O2, H2S, pH and Eh), geochemical and mineralogical methods, scanning transmission electron microscopy (STEM), omics tools (metagenomics, metatranscriptomics, metaproteomics, metabolomics) and multispecific and axenic microbial cultures. The scientific and socioeconomic impact of BIOCOBRE, if we are able to achieve Cu0 precipitation, could be high. It could lead to patents and would probably open a new line of research focused on the bioengineering of the Cu0 precipitation process, to make it a technically and economically viable alternative to recover Cu0 from AMD, using an ecological approach at a reduced cost, in which Andalucia would be at the forefront.
The project (P20-01048) is co-financed by the European Union, within the framework of the Andalusia FEDER Operational Program “Smart growth: an economy based on knowledge and innovation, also responding to the Research and Innovation Strategy for the Smart Specialization of Andalusia (RISˑAndalusia) and the priorities and objectives set out in the Andalusian Plan for Research, Development and Innovation (PAIDI 2020).
Researchers from different departments and universities collaborate in the project:
Corzo Rodriguez, Alfonso, Dept Biología, Area de Ecología. Investigador principal, Coordinación general. Ecología microbiana
Papaspyrou, Sokratis, Dept Biología, Area de Ecología. Ecología microbiana.
García Robledo, Emilio Guillermo, Dept Biología, Area de Ecología. Ecología microbiana.
Duran Ruiz, Maria Del Carmen, Dept Biomedicina, Biotecnología y Salud Publica, Area de Bioquímica. Metaproteonómica.
Garrido Crespo, Carlos, Dept Biomedicina, Biotecnología y Salud Publica, Area de Microbiología. Aislamiento de microorganismos.
Lajaunie, Luc Cyrille Jacques, Dept de Ciencia de los Materiales e Ingeniería Metalúrgica y Química Inorgánica. Microscopia electrónica y análisis mineralógicos
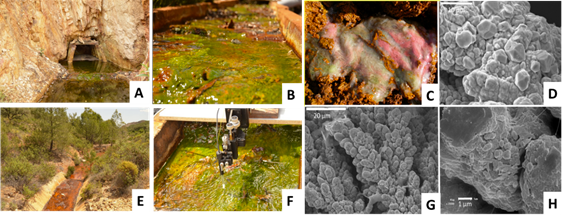
Fig. 1. Exit of the AMD of Mina Esperanza (Huelva) and precipitation channel (A, E). Biofilm surface in the channel (B). O2 microsensor coupled to a micromanipulator measuring in situ (F). Portions of the biofilm with accumulations of precipitated Cu (pink color) (C). SEM images of Cu precipitation within the pink masses at different magnifications (D, G, H). Precipitated Cuº is found in close association with amorphous and filamentous structures that could represent the EPS matrix of the biofilm and bacterial structures such as nanowires (H).
Also participating in the project are:
Castillo Hernández, Julio Cesar. Univeridad de Free State, Sudafrica. Geomicrobiología, herramientas moleculares y microscopia.
Valverde Portal, Angel, IRNASA-CSIC. Microbiología.
Taylor, Joe Daniel, UK Centre for Ecology & Hydrology. Metagenómica.
The project (P20-01048) is co-financed by the European Union, within the framework of the Andalusia FEDER Operational Program “Smart growth: an economy based on knowledge and innovation, also responding to the Research and Innovation Strategy for the Smart Specialization of Andalusia (RISˑAndalusia) and the priorities and objectives set out in the Andalusian Plan for Research, Development and Innovation (PAIDI 2020).
Participation in the SR2114 cruise
CEIMAR Young researcher’s project (2019 call)
One of our post doc members, who is associated to the project MICROBAHIA and MICROBAHIA 2, as well as an active researcher, Dr. Miguel Lara, has been successful in obtaining financial support by the CEIMAR foundation.
Miguel Lara’s project is titled “Oxygen dynamics in phototrophic intertidal sediments. Stoichiometry of proton fluxes and effects of porewater drainage”. The main goal of his proposal is to clarify the influence of tidal state in the short term response of oxygen and pH gradients, both due to microphytobenthic activity and diffusive fluxes. For such objective, our laboratory benefits from a fully developed equipment of microsensors and an additional budget of 4000 €.
Due to the coronavirus pandemic disease (COVID-19), CEIMAR foundation extended the end date of the project along 2021.
More info can be found here:
https://www.researchgate.net/project/CEIMAR-Program-for-young-leading-researchers-2019