Aquatic Eddy Covariance (EC) is a powerful new technique used to measure O₂ exchange, between water and the sediment below. This provides measurements of the net metabolism of the sediment and the primary production which are used to estimate how much CO₂ is absorbed or released by underwater environments.
How Aquatic Eddy Covariance Works
Aquatic Eddy Covariance (AEC) allows to measure the fluxes of O₂ between sediment and water. The principle behind AEC is based on capturing turbulent movements (or “eddies”) of water, which carry dissolved gases between the sediment and the overlying water column. Here’s a closer look at how it operates:
What are the Eddies
In natural aquatic environments, water constantly moves due to currents, waves, and turbulence. These small, swirling currents, called eddies, bring water in contact with the sediment surface, where various biological and chemical processes take place that consume or produce oxygen, altering the concentration of O2.
If the sediment is under light and there are photosynthetic organisms that produce O2, they will release it to the overlying water column increasing its concentration in comparison to water that is higher up in the water column. Thus, an eddy might transfer water with higher concentration to an area with lower concentrations. In contrast, in darkness, all teh heterotrophic organisms in the sediment consume O2, the sediment will act as a sink, and the concentrations of O₂ closer to the sediment will be lower than higher up in the water column. Thus, the concentration of O2 at certain height in the water column varies within the eddies depending on the interactions with the sediment below.
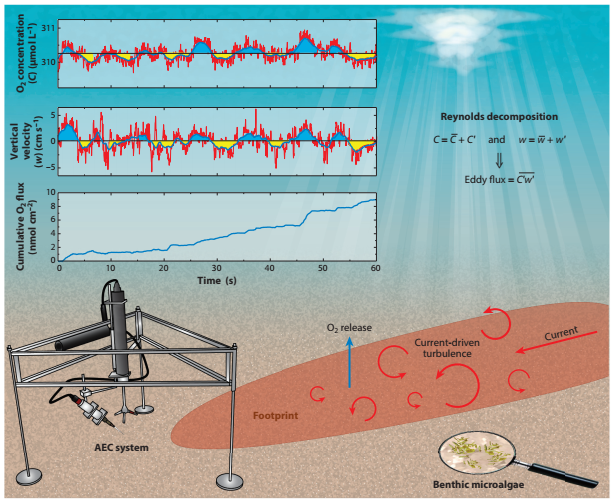
“An AEC system (lower left) collecting data over a light-exposed seafloor that releases O2 due to photosynthesizing benthic microalgae (lower right). The seafloor area producing the eddy flux signal is referred to as the footprint and is located upstream from the system (red shading). Three graphs (upper left) show 1 min of fast measurements (8 Hz) of the fluctuating O2 concentration (C, top), the fluctuating vertical velocity (w, middle), and the cumulation of the instantaneous flux (bottom). During periods marked with yellow, the vertical velocity is directed down, and the O2 concentration is below average. During periods marked with blue, the velocity is directed up, and the O2 concentration is above average. This highly dynamic but persistent turbulent pattern will, when averaged over time, give a net transport of O2 up from the benthic substrate. The linear trend of the cumulative O2 flux indicates a strong flux signal in the data. Abbreviation: AEC, aquatic eddy covariance. AEC system drawing by Luke Cole, University of Virginia.”
Sensor Setup
The AEC setup typically includes:
- An Oxygen microensor: The method requires a fast-response sensor that can detect the concentration of the target gas in the water in msec. It should be highly sensitive and detect rapid changes in concentration within each passing eddy.
- A 3D Acoustic Doppler Velocimeter (ADV): This measures the speed and direction of water flow in 3 dimensions, capturing the motion of eddies moving past the sensor.
These two sensors have to be mounted close to the sediment surface, positioned so they can “see” the same parcel of water almost simultaneously. This configuration enables the system to detect both the concentration of gases and the movement of water in three dimensions.
Data processing and Analysis
The AEC system continuously measures both the water flow and gas concentration at a high frequency (often multiple measurements per second). By synchronizing these two sets of measurements, we can analyze the data to determine how much O2 is being transported by each eddy as it moves upward or downward on the vertical axis.
To calculate the gas flux, we have to use a statistical approach called “covariance analysis.” Essentially, we have to examine the correlation between the water’s vertical movement (upward or downward) and changes in gas concentration. When upward-moving eddies have a higher concentration of oxygen, for example, it indicates that oxygen is being released from the sediment into the water. Conversely, if downward eddies carry more O₂, the system can detect that O₂ is being consumed by the sediment.
AEC footprint
The area covered by AEC depends primarily on the water flow, sensor height above the sediment, and the turbulence characteristics of the environment. Generally, AEC can capture flux measurements over areas of about 10 to 100 square meters in typical shallow water environments.
Some factors that affect the footprint include:
- Water Flow: Faster flows tend to expand the area (or footprint) that contributes to the flux measurement.
- Sensor Height: Placing the EC sensor higher above the sediment surface increases the footprint size but may reduce the accuracy in environments with complex water flows.
- Environmental Conditions: High turbulence and variable flow direction, often found in tidal areas, can modify the effective footprint and the averaging time needed for reliable data.
Real-Time and Integrated Measurements
Aquatic EC operates continuously, providing real-time data that reflects the natural, unaltered environmental conditions. It captures fluctuations in gas exchange throughout the day and night, offering insights into diurnal cycles and how processes respond to changing environmental factors such as light, temperature, and water flow.
Benefits of Aquatic Eddy Covariance Over Traditional Methods
AEC provides several significant advantages over traditional methods, such as benthic chambers or whole core incubations.
Natural, In-Situ Measurements
AEC captures fluxesin the natural, undisturbed environment, preserving typical water flow, light, and temperature conditions. This approach avoids the confinement effect found in traditional methods like benthic chambers, where conditions are often altered, leading to less accurate results.
High Temporal Resolution
AEC records continuous data at high frequencies, allowing for detailed, real-time tracking of fluxes. This high temporal resolution reveals patterns throughout day-night cycles, seasonal changes, and responses to environmental events (like storms or temperature fluctuations) that may be missed with periodic sampling.
Broad Spatial Coverage
AEC measures fluxes over a larger area (10-100 square meters) as mentioned, integrating information across various environments. This broad coverage provides a more comprehensive view of ecosystem processes than single-point measurements, even when several replicates are used.
Sensitive to Environmental Changes
AEC is highly responsive to environmental changes like shifts in water flow, light availability, and nutrient inputs, enabling precise detection of how these factors impact fluxes. This sensitivity is crucial for understanding the effects of climate change on coastal and marine ecosystems.
Non-Invasive Monitoring of Benthic Ecosystems
Since AEC measures eddies and gas concentrations without physically disturbing the sediment, it is minimally invasive. In addition, it can be used in both soft sediment and hard substrate areas, where benthic chambers cannot be used without damaging the habitat. This feature makes it ideal for studying sensitive habitats, such as seagrass beds, coral reefs, and mudflats, where disturbance could impact the organisms and processes being studied.
Efficient Long-Term Monitoring
AEC is well-suited for long-term installations, providing a continuous data stream over extended periods. However, the sensors have to be cleaned regularly to prevent biofouling that would affect the sensitivity of the sensors.
In summary, AEC offers a unique and highly effective approach to studying aquatic ecosystems, providing real-time, detailed, and accurate data on gas exchange. These benefits make it invaluable for advancing our understanding of aquatic ecosystem dynamics, resilience, and contributions to global carbon cycling.